It really is. But why?
This article will, I hope, give you some idea why. What it won’t do is catalogue the different types of DRI you might encounter and what the requirements are to handle or carry them safely. That might be a future article, but this one is meant to explain the science underlying DRI and what gives rise to its hazards.
Iron is a very familiar substance – we make all sorts of things out of it or things based on it. Steel structures are found in buildings, cars are made from steel, we have iron railings and gates, and there are many many more examples. There’s a property in common with many of these man made objects, and that is that they rust if you don’t prevent it. Rusting is a very familiar example of an oxidation reaction. Iron plus oxygen becomes iron oxide, one type of which is the familiar red/brown rust we see on iron railings and the steelwork of older cars.
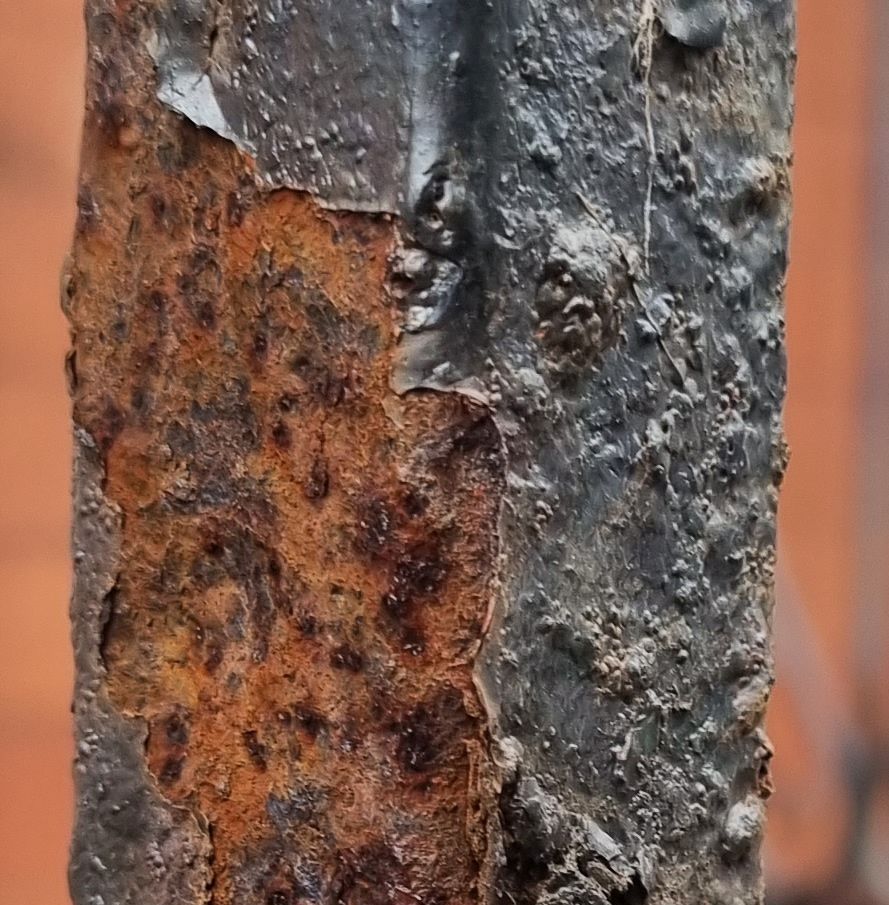
There is lots of iron around in the earth’s crust – that is because the familiar isotopes of iron are, in nuclear physics terms, the most stable substances known. Nuclear stability arises from the lack of tendency to undergo nuclear fission or nuclear fusion. Those two processes release energy from the nucleus when that nucleus splits (fission) or merges with another (fusion). Splitting a nucleus of iron will always cost energy, similarly trying to fuse iron with other nuclei will also absorb energy. Thus iron nuclei are highly stable, and form a substantial proportion of the end-products of the explosions known as supernovae and in other stellar processes. As these are where the elements making up the earth (including those in you and me!) are formed, the presence of large amounts of iron (and also nickel) in the earth’s crust and core is unsurprising. But I digress.
Iron is a moderately reactive metal and is always found in nature combined with other substances as ores. Iron ore might contain iron oxides, iron sulphides, or other forms.
Production of metallic iron from naturally occurring iron ores was first carried out by mankind over 3000 years ago. It was such an important development that the term “iron age” describes the period when iron became available for use. Early furnaces could not reach the melting point of iron and produced a form of the metal known as a “bloom”. Later furnaces used forced air draughts to reach higher temperatures and are known as blast furnaces. These produce molten iron, known as “pig” iron which can then be used in steelmaking.
Ironically (geddit?) DRI has more in common with obsolete bloom iron than more modern blast furnace forms. Consider an ore which is mostly iron oxide. Chemically, this is an example of an ionic material. The iron atoms are present as positively charged iron ions, and the oxygen atoms are present as negatively charged ions. In a piece of solid iron ore, between each pair of adjacent iron atoms is found an oxygen atom, and vice versa.
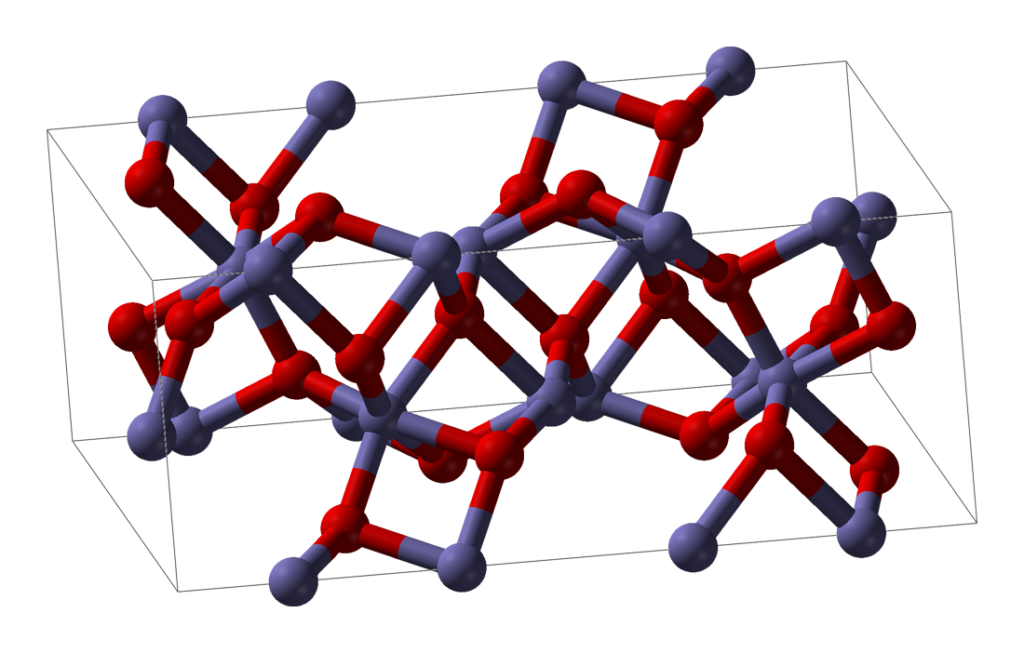
Direct reduction involves the removal of the oxygens without melting the feedstock. Typically the feedstock is pelletised iron ore, each pellet being roughly 1cm in diameter. When the oxygen is removed from the pellet without melting the pellet, the shape and size is retained but the locations which used to have oxygen atoms in them now have gaps. These tiny gaps are all interconnected and the resulting pellet of DRI is extremely porous. It has a huge surface area yet weighs less than the pellet it was made from. Once reduced, it looks very much like a malteser (without the chocolate coating).
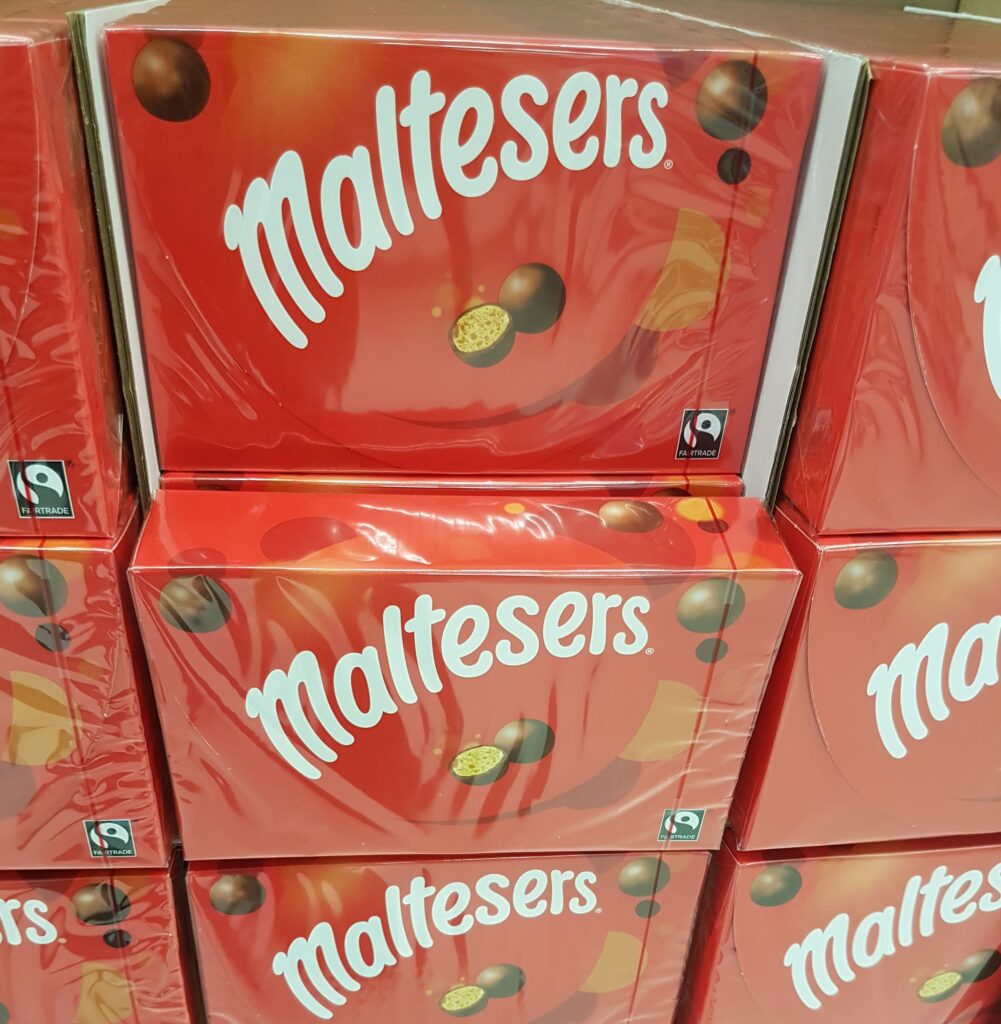
I’m going to refer to DRI made by the MIDREX process, which is made in a shaft furnace. The reducing agent (i.e. the material used to remove the oxygen) is hydrogen and carbon monoxide made from natural gas. Natural gas is also used to heat the furnace, but because the ore is not melted, less heat is required than other furnace types, meaning that the DRI process is relatively energy-efficient. The need for natural gas to heat the furnace and provide the reducing gas means that DRI plants are usually found in locations where gas is plentiful and relatively inexpensive.
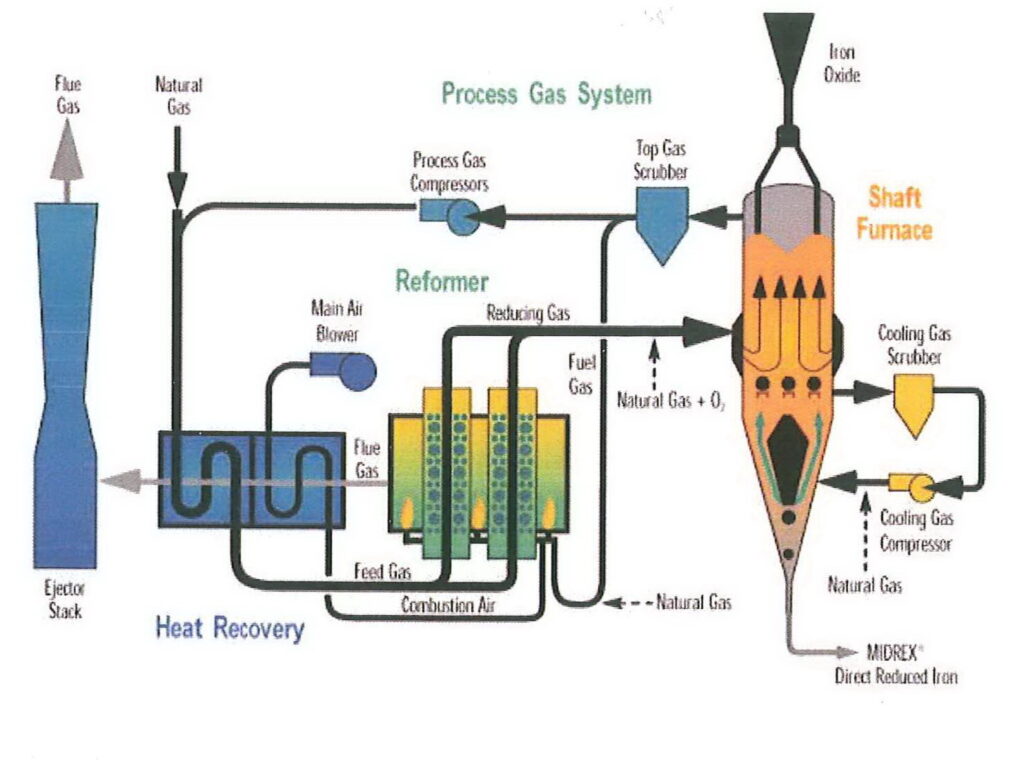
A parameter often quoted in the context of DRI is % metallisation. This is the percentage of the iron in the pellet which is present as free metallic iron rather than oxides. With modern DRI facilities, metallisation will be 95% or so. This iron can be used directly in steelmaking.
Because DRI production tends to take place where the natural gas is, it is often then carried by sea to a steel mill. That is unfortunately where some of the problems arise.
All of the problems associated with DRI come about because of the physical form that iron is in. A lump of iron can rust at its surface. That rusting usually involves water, and rusting by sea water is more rapid than with fresh water. Rusting to a cargo of DRI, especially if wetted by sea water ingress, can damage the cargo by allowing parts of it to revert to iron oxide. Loss of cargo value is undoubtedly something a shipowner will want to avoid, but there are much more immediate problems a vessel is likely to face if DRI becomes damaged on board.
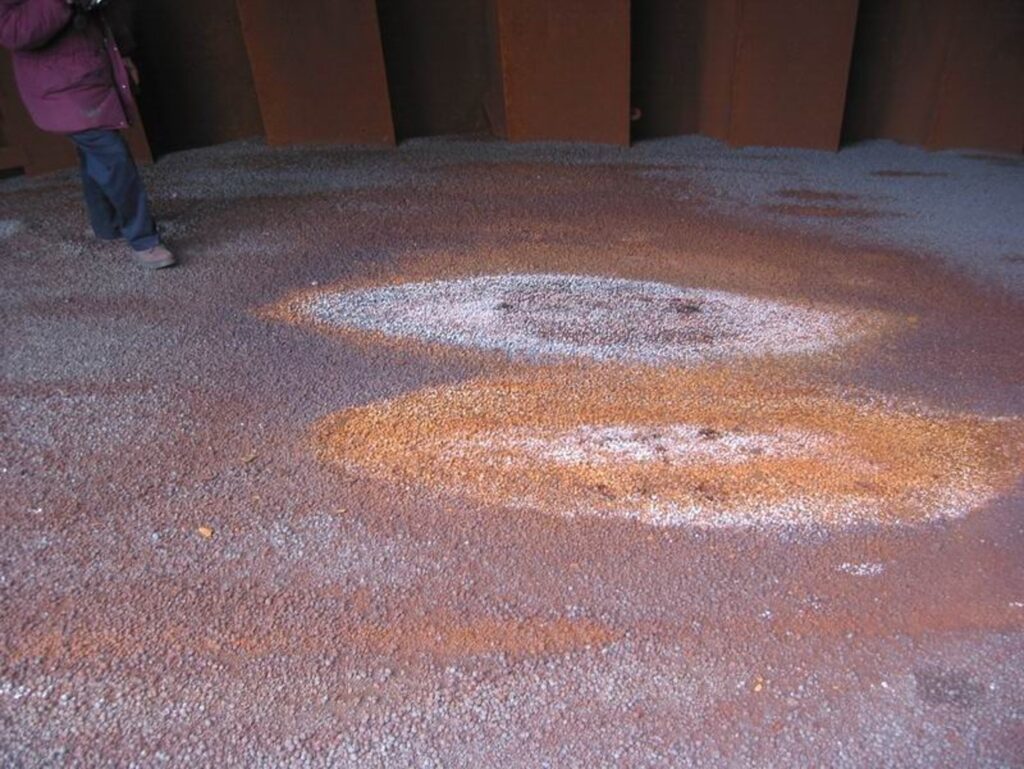
Rusting is an oxidation reaction, and like all oxidative reactions, involves the release of heat. Quite a lot of heat in chemical terms, but we don’t notice a rusty car or iron railing getting hot as it rusts. Why not?
The answer is that the rusting only happens at the exposed surface of the railings or car structure, and does so slowly enough that any heat generated is immediately lost to the atmosphere. There’s never a temperature rise.
In contrast, a pellet of DRI has a huge surface area – some estimates suggest that a pellet of DRI has a surface area 10000 greater than a lump of solid iron. That means that when the DRI is freshly produced it has 10000 times the capability of reacting with oxygen and rusting. That’s one reason that DRI behaves differently to a lump or a gate made of iron.
The other hugely significant property of DRI is that, like most bulk cargoes, it is a poor conductor of heat. That might be a little surprising to hear as there is nothing there other than iron, and metals conduct heat, don’t they?
A block of iron does indeed conduct heat. A bulk made up of spheres made out of iron does not conduct very well because the contact area between adjacent spheres is small and thermal contact is poor. A bulk made up of DRI, in which much of the surface area is space rather than metal conducts heat very badly indeed.
That means that where (for example), sea water enters a bulk stow of DRI, the first thing which happens is that the wetted iron rusts. Fresh water will cause rusting but sea water makes it happen much more rapidly. The heat released by the rusting reaction, which for most of the forms of iron we encounter everyday is lost immediately, does not get conducted away and makes the temperature rise locally where the DRI was wetted.
As the temperature of the iron at those points within the stow rises, it may reach a high enough temperature that the iron can react directly with the oxygen in the hold (if there is any!). This isn’t rusting any more but is direct oxidation. The higher the temperature gets, the more rapidly the reaction goes.
If there is a ready supply of fresh air/oxygen, a DRI cargo can heat to several hundred degrees Celsius and will keep going. Whilst the rate of heat conduction within the bulk is low, it isn’t nil, and gradually the volume of DRI involved with what is now referred to as a “metal fire” increases. It may pass from hold to hold through a bulkhead. It may overheat fuel in adjacent tanks.
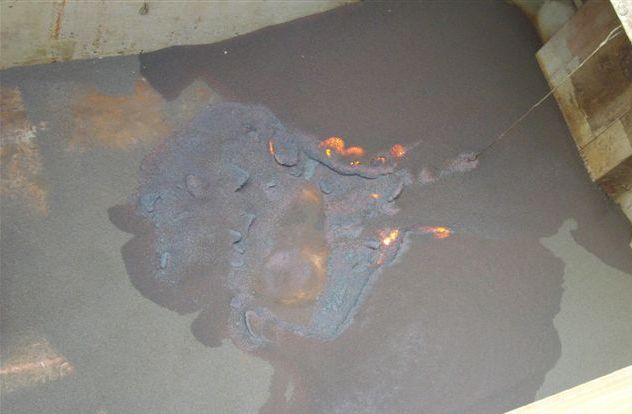
As I said, this article is not going to go into detail about what the different types of DRI are and how they should be carried. I will say, however, that one of the main tools in the arsenal of a vessel carrying DRI is the ability to exclude oxygen from the stow. This may be enough to prevent a fire in the first place, or it may help in keeping a situation under control so the vessel can safely reach a port where the problem can be dealt with. Nitrogen gas is used to inert holds carrying some types of DRI precisely for this purpose. Unfortunately, the more commonly found carbon dioxide inert gas is not suitable.
When DRI gets to high temperatures, it becomes a very difficult commodity to deal with. Hot DRI really loves oxygen, and will do almost anything to get it. Nitrogen is effective in stabilising where the hatches are closed, but oxygen will inevitably get into the stow during discharge. Temperatures can get very high indeed and there can be a risk to the vessel itself.
The only recourse available to assist during the discharge of a problem DRI cargo is to use water. Even that is fraught with difficulty. There are multiple problems. Firstly, problematic DRI can be at several hundreds of degrees or more. Initial amounts of water will simply be boiled off as steam. You have to be able to put water in there quicker than the DRI can boil it off.
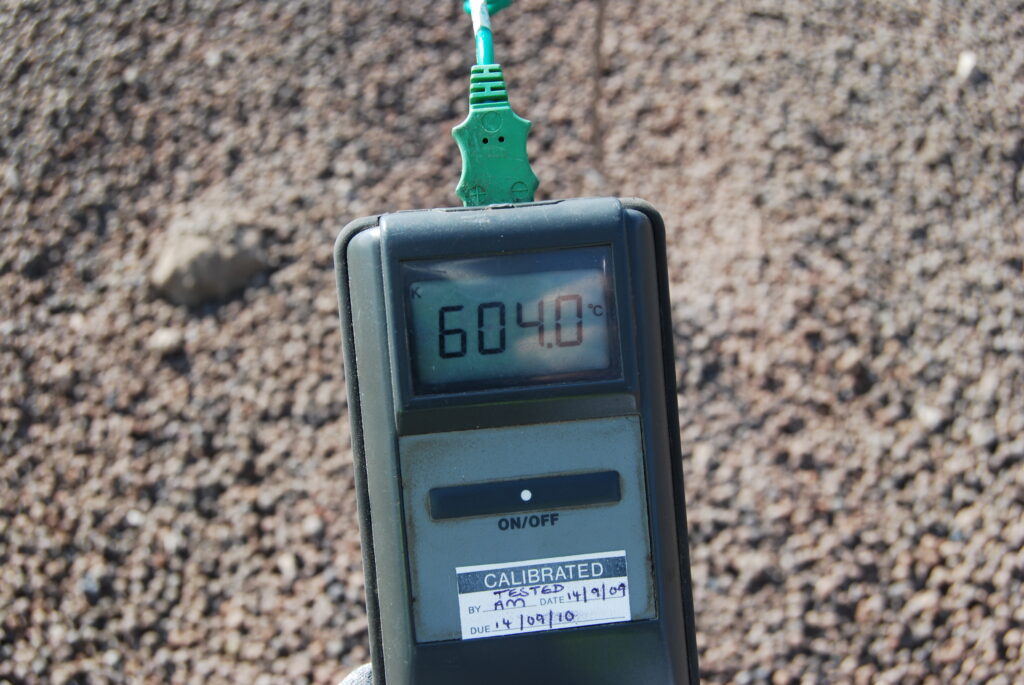
Next problem is that water is H2O, and that O is of course oxygen. Hot DRI can satisfy its need for oxygen directly from the water you are pouring on it. The DRI takes the O, creating more heat and leaving the H2. This is hydrogen gas, very volatile and highly flammable. Be ready for whooshes of flame from the hydrogen, assuming you successfully avoid an explosion.
And then there is the fact that you are putting water, possibly even sea water, onto DRI. Any thought you might have had of salvaging any of the cargo is probably history by now, but there is a very real problem of creating further heating in stockpiles (hopefully ashore) from wetting by extinguishing water.
If all of that wasn’t enough, the final insult is that that the resulting oxidised DRI will weigh about 30% more than the DRI cargo you started with. Anecdotally, problems involving the collapse of quay structures have been caused by underestimating the weight of the reoxidised cargo.
Phew. If you are going to carry DRI, it really is very important that you don’t have a problem. But DRI can cause difficulties even when there isn’t an emergency/fire situation. What might they be?
Inevitably during loading and discharge, dust is created. That dust is metallic iron. The IMSBC Code warns about the need to remove accumulations of dust as quickly as possible. I have seen first-hand what happens if you don’t or can’t. Accumulations of DRI dust embed themselves into paint and then rust. This is not only unsightly but it can cause significant operational problems. The Code also warns about the potential for damage to radio and other aerials/antennae.
All in all, carriage of DRI is not something to be embarked upon without a great deal of preparation and care. At Sheard Scientfic, we have first-hand experience of dealing with carriage and consequences of carriage of DRI and can assist our clients with this highly problematic material.